Lasers: 3 Surprising Types, Applications, And Devices That Use Them

By John Oncea, Editor
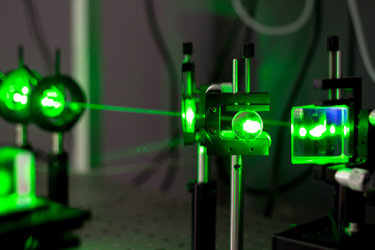
Let’s look at three unique types and applications of lasers, as well as three devices that use them - you won’t believe number three.
Lasers come in all shapes and sizes including gas lasers, diode lasers, semiconductor lasers, dye lasers, fiber lasers, pulsed layers, and liquid lasers.
Lasers have many uses including in science, medicine, communications, chemistry, printing, data storage, imaging, welding, robotics, surveying, mapping, guidance, and cutting.
Lasers are used in many devices including printers, barcode scanners, laser light pointers, light show projectors, optical tweezers, laser welders, 3D scanners, and mobile devices.
But you knew all of that, didn’t you? Let’s see if we can come up with three types, applications, and ways lasers are used that will surprise you.
What Is A Laser?
We’ve written about lasers before but, c’mon, they’re so cool! The more you dig into them the more interesting they become. That said, before we get into surprising types, applications, and devices, let’s take a 40,000-foot look at lasers.
A laser (an acronym for Light Amplification by Stimulated Emission of Radiation), “is created when electrons in the atoms in optical materials like glass, crystal, or gas absorb the energy from an electrical current or a light. That extra energy ‘excites’ the electrons enough to move from a lower-energy orbit to a higher-energy orbit around the atom’s nucleus, writes Lawrence Livermore National Laboratory. “A laser takes advantage of the quantum properties of atoms that absorb and radiate particles of light called photons. When electrons in atoms return to their normal orbit — or “ground” state — either spontaneously or when stimulated with a light or other energy source, even another laser in some cases, they emit more photons.”
When light waves in a laser beam move in the same direction and wavelength, we call them “coherent.” This is achieved by passing energized electrons through an optical “gain medium,” which could be a solid material like glass or a gas. The wavelength of light is determined by the amount of energy released when an excited electron falls to a lower orbit. The levels of energy can be adjusted in the gain medium to produce the desired beam color.
A mirror is placed on one side of the optical material of the laser. This mirror reflects the photon to the electrons. The space between two mirrors, also called the “cavity,” is designed in such a way that the desired photon, required for the specific type of optical gain medium, is fed back into the medium to trigger the emission of an almost exact copy of that photon. Both the original photon and its clone move in the same direction and speed, and then they bounce off another mirror on the opposite side to repeat the cloning process.
“Two become four, four become eight, and so on until the photons are amplified enough for them to all move past the mirrors and the optical material in perfect unison. Think of them as syncronized members of a marching band in the Rose Parade. And that unison gives the laser its power. Laser beams can stay sharply focused over vast distances, even to the moon and back.”
The names associated with establishing theoretical foundations, developing, and refining lasers read like a who’s who of science. Einstein, Kastler, Bohr, Townes, Basov, Prokhorov, Nishizaea, Schawlow, Gould, … the list goes on and on.
Each of these scientists built on the previous scientist’s breakthrough over the years, culminating in 1960 when Theodore Maiman operated the first functioning laser at Hughes Research Laboratories, Malibu, CA. Maiman’s device was only capable of pulsed operation but later that year Ali Javan, William Bennett Jr., and Donald Herriott constructed the first gas laser using helium and neon that was capable of continuous operation in the infrared.
Two years later, Robert Hall demonstrated the first semiconductor laser which was made of gallium arsenide and emitted in the near-infrared band of the spectrum at 850 nm. Later that year, Nick Holonyak demonstrated the first semiconductor laser with a visible emission. In 1970, Zhores Alferov in the USSR and Izuo Hayashi and Morton Panish of Bell Labs also independently developed room-temperature, continual-operation diode lasers, using the heterojunction structure.
Bada bing, bada boom, the laser.
Surprising Types
Lasers are most commonly classified based on the optical gain medium which can be either solid, liquid, or gas, according to xTool. They also can be classified based on how the laser beam radiates out, commonly known as the mode of operation. There are two primary types: continuous-wave lasers and pulsed lasers. Finally, lasers can be classified by the wavelength of light it emits based on where it falls on the electromagnetic spectrum. This included ultraviolet lasers (UV), visible lasers, and infrared lasers.
Some common types of lasers are fiber lasers, gas lasers, semiconductor lasers, and pulsed lasers. But we’re looking for surprising types of lasers, so I present to you spasers, neodymium lasers, and gamma-ray lasers.
Let’s start with spasers (an acronym for surface plasmon amplification by stimulated emission of radiation) which are effectively nanoscale lasers with subwavelength dimensions and low-Q plasmonic resonators that sustain their oscillations using stimulated emission of surface plasmons, according to Optica.
A spaser aims to confine light at a subwavelength scale, far below the Rayleigh diffraction limit of light and achieves this by storing some of the light energy in electron oscillations called surface plasmon polaritons (SPPs). Unlike conventional lasers that emit photons, a spaser replaces photons with SPPs and the resonant cavity with a nanoparticle that supports plasmonic modes.
The energy source for spasing is an active (gain) medium that gets externally excited. For instance, a spaser can operate in the near-infrared, while its gain medium can be excited using an ultraviolet pulse.
Surface plasmons in a spaser behave analogously to photons in a laser due to their similar physical properties:
- Bosonic Nature: Like photons, surface plasmons are vector excitations with spin.
- Electrically Neutral: Surface plasmons are electrically neutral excitations.
- Collective Oscillations: They are the most collective material oscillations known, interacting weakly with each other.
Spasers can undergo stimulated emission, accumulating in a single mode, similar to the physical foundation of both lasers and spasers. Researchers envision various applications for spasers, including nanoscale lithography, ultra-fast photonic circuits, single-molecule biochemical sensing, and microscopy.
Next up, neodymium lasers – often referred to as Nd:YAG lasers – which play a crucial role in various technological advancements, offering versatility, efficiency, and precise laser output.
“Neodymium lasers (Nd lasers) are compact, long life, and robust, making them suitable for various applications: industrial integration, research laboratories, and portable applications,” writes Xometry. “They are used in medical intervention in dermatology and ophthalmology, for procedures such as tattoo removal, skin resurfacing, hair removal, and treatment of vascular lesions. Neodymium lasers are often used as the pump source for other laser types.”
Nd lasers are a type of solid-state laser that uses neodymium-doped crystalline materials as the active medium. These lasers are designed to emit a range of spectra by varying the host crystal, with most Nd lasers producing 1.064 µm (IR). By adding a frequency doubler or a parametric oscillator, the beam can be retuned to emit green, blue, and ultraviolet radiation.
Nd lasers are highly efficient and deliver high-energy radiation, making them ideal for applications such as laser cutting, welding, and laser marking. They can be operated in continuous wave (CW) or pulse mode. CW operation is best suited for material processing, while pulsed Nd lasers are used in applications that require precise temporal control, such as laser-induced breakdown spectroscopy, range finding, and laser ablation.
Finally, gamma-ray lasers – also known as a graser – which is a theoretical device that would produce coherent gamma rays similar to how an ordinary laser generates coherent visible light rays. These high-energy gamma rays have numerous potential applications, including:
- Medical Imaging: Gamma-ray lasers could enhance medical imaging techniques, providing detailed insights into tissues and organs.
- Spacecraft Propulsion: Imagine a spacecraft powered by gamma-ray lasers, enabling faster and more efficient space travel.
- Cancer Treatment: Targeted gamma-ray lasers might revolutionize cancer therapy by precisely destroying cancer cells.
However, it’s essential to note that grasers do not currently exist. Researchers continue to explore the challenges associated with creating practical gamma-ray lasers, involving interdisciplinary fields such as quantum mechanics, nuclear physics, and materials science.
Surprising Uses Of Lasers In Science, Defense, And Music
Well, you know barcodes and LiDAR, and optics and cutting, but do you recall, surprising lasers at all? Well, here I three uses I came up with, one each related to science, defense, and music.
When the Apollo 11, 15, and 15 astronauts – as well as the Soviet Lunokhod 1 and 2 missions and India’s Chandrayaan-3 mission – visited the Moon, a total of six retroreflector arrays were placed on the lunar surface and the Lunar Laser Ranging (LLR) experiment was created. Laser beams are focused through large telescopes on Earth aimed toward the arrays, and the time taken for the beam to be reflected to Earth is measured to determine the distance between the Earth and the Moon with high accuracy.
Although it is possible to reflect light or radio waves directly from the Moon's surface (a process known as EME), a much more precise range measurement can be made using retroreflectors, since because of their small size, the temporal spread in the reflected signal is much smaller.
The LLR experiment has provided evidence that supports Albert Einstein’s general theory of relativity and helped researchers understand changes in Earth’s rotation and continental drift. They also have made several discoveries, including:
- The Moon is spiraling away from Earth at a rate of 3.8 centimeters per year
- The Moon has a fluid core
- The Moon has tides in its rock that displace the surface
- The Moon’s orientation can be measured
On to defense and the laser dazzler, a non-lethal weapon that uses intense directed radiation to temporarily disorient its target. Dazzlers can effectively deter further advances, regardless of language or cultural barriers and are used for hailing, warning, and crowd control. Targets include both electronic sensors (such as cameras or sensors) and human vision.
Dazzlers emit infrared or invisible light against electronic sensors but against humans, they emit visible light and are designed not to cause long-term damage to the eyes. These systems can operate in either the red (using a laser diode) or green (using a diode-pumped solid-state laser, DPSS) areas of the electromagnetic spectrum, and the green laser is chosen for its unique ability to react with the human eye.
Dazzlers maintain eye safety by producing diverging light that is less focused than typical lasers resulting in a larger, less concentrated spot at greater distances making it easier to aim and retain the desired effect on targets without causing harm.
Laser dazzlers were first reported in combat during the Falklands War in 1982 and, in more recent times, the U.S. military used laser dazzlers mounted on M4 rifles in Iraq to non-lethally stop drivers who failed to halt at checkpoints
Finally, lasers in music – specifically the laser harp. According to Laser Harp, a laser harp is an electronic musical instrument consisting of several laser beams to be blocked, in analogy with the plucking of the strings of a harp, to produce sounds.
Laser harps are typically built in either a Framed or Unframed style. “Framed style laser harps are built to resemble a classical harp with strings. Photodiodes, as well as low-powered lasers (usually around 5mW), are set inside a frame,” Laser Harp writes. “The number of lasers can vary from a simple harp with one or two lasers up to 32 or more, depending on the complexity of MIDI hardware and software.
“Unframed style harps usually consist of three components – laser projector, controller, and a sensor. The first versions of unframed laser harps had all three elements set in a single box. Recently, however, laser harps are built in a way that the three components can be placed independently and connected by proper cables.”
A laser projector and a sensor are positioned in a way that they face each other, and both of them are connected to a controller box. This setup makes the system more versatile, allowing you to use any type of laser projector with power ranging from 5 to 20 watts or even more. Additionally, you can place the components in any configuration you desire. The controller box functions as a MIDI controller, which sends MIDI signals. Unframed laser harps are more complex to construct than framed laser harps, but they provide greater flexibility and adaptability.
A laser harp projects an array of beams in a fan arrangement. When one of the laser beams is blocked, a sensor detects it and sends a signal to the Laser Harp Controller. The controller then sends a MIDI signal to a synthesizer, sampler, or PC sound card. This device is responsible for playing the desired sound according to the information received through the MIDI signal.
“Benefits from using high-powered lasers are multiple since they make it easier for the sensor to detect an interrupted laser beam and they provide a more compelling visual experience for the audience,” adds Laser Harp. “Laser harp performers usually wear white gloves to improve the sensor’s ability to pick up the reflected light and as a protection from potentially hazardous laser radiation as well as to give an audience a more impressive visual performance.”
Surprising Devices
So, you’re going to have to bear with me on this one because sharks aren’t devices. But here we go. Lasers are part of DCD and CD players, as well as laser pointers (duh). But they are also components in speech-jamming guns, Googling, and armed sharks.
A speech jammer is a device that leverages Delayed Auditory Feedback (DAF), an auditory phenomenon that disrupts a person’s ability to continue speaking fluently by echoing their voice back to them with a slight delay.
“The idea is based on the fact that to speak properly, we humans need to hear what we’re saying so that we can constantly adjust how we go about it, scientists call it delayed auditory feedback,” Phys.org writes. “It’s partly why singers can sing better when they wear headphones that allow them to hear their voice as they sing with music or use feedback monitors when onstage.
“Trouble comes though when there is a slight delay between the time the words are spoken and the time they are heard. If that happens, people tend to get discombobulated and stop speaking, and that’s the whole idea behind the SpeechJammer. It’s basically just a gun that causes someone speaking to hear their own words delayed by 0.2 seconds.”
This is accomplished by attaching a directional microphone and speaker to a box that also holds a laser pointer, distance sensor, and computer board to compute the delay time based on distance from the speaker. The person using it points the gun at the person talking, using the laser pointer as a guide, then pulls the trigger. It works for distances up to a hundred feet.
Next up, Google, which is using laser tech to bring cheap internet to remote areas. According to Euro News, Google has “turned to a new technology for what they call the Taara project: using neatly designed terminals that beam data carrying-lasers to corresponding terminals over fixed distances – essentially fiber-optic internet without the cables.”
Tarra engineers are experimenting with mirrors of different focal lengths and special tables designed to recreate real-life conditions such as shaking from the wind, animals, or traffic that terminals would be subjected to out in the field.
And finally, sharks with frickin’ lasers. Marine biologist Luke Tipple once attached a 50-milliwatt green laser to a lemon shark's dorsal fin via a “non-invasive clamp,” Wired writes. “Tipple says he chose a lemon shark – Negaprion brevirostris – for its ‘predictable and relatively docile swimming behavior during the day, ease of access in shallow water, and size of the dorsal fin.’”
To put it simply, the shark used in the stunt was small enough to be easily contained and would not swim too far away. As expected, Tipple’s team successfully retrieved the shark used in the experiment and removed the clamp.
Tipple said the experiment allowed him to evaluate his clamping apparatus, which is typically used for traditional data-acquisition equipment, as well as verify anecdotal evidence that sharks avoid laser energy of specific spectrums and wavelengths. “Curiously,” Wired writes, “he found the opposite to be true: ‘Although further testing is necessary, time and time again, sharks were attracted to the laser beam,’ he said.”
The experiment also helped measure the shark’s velocity and trajectory in real time. “We were able to see how their body positioning relates to a target,” Tipple said. “You can get a very clear description, via the laser, of what the shark’s body is doing.”