DE, LEO, And 3D: 3 Unique Applications Of Laser Technology

By John Oncea, Editor
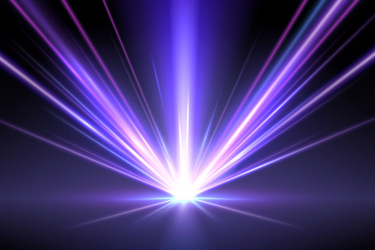
Lasers are used in many ways across nearly every industry. Here, we take a look at how they are being used by the military and in LEO, as well as future use in medical electronics.
Lasers is the third studio album by American rapper Lupe Fiasco, released on March 7, 2011, by Atlantic Records. Hold on … I’m being told that’s the wrong lasers.
The lasers* we’re interested in, according to Lawrence Livermore National Laboratory, are “created when electrons in the atoms in optical materials like glass, crystal, or gas absorb the energy from an electrical current or a light. That extra energy ‘excites’ the electrons enough to move from a lower-energy orbit to a higher-energy orbit around the atom’s nucleus.”
Albert Einstein first conceptualized laser technology in 1917 when he predicted the phenomenon of “Stimulated Emission.” Forty-three years later, they became a reality when Theodore Maiman built the first working prototype of a laser at Hughes Research Laboratories in Malibu, CA. “This laser uses synthetic ruby as the active medium and emits a deep red beam of light with a wavelength of 694.3 nm,” writes Universal Laser Systems. “The first application for the ruby laser was for military range finders and is still used commercially for drilling holes in diamond because of its high peak power.”
Lasers are used for a variety of applications in many disparate industries. Let’s take a look at three specific uses of lasers: as part of directed energy (DE) weapons, for communications in low earth orbit (LEO), and in 3D printing.
* Did you know “laser” is an acronym for Light Amplification by Stimulated Emission of Radiation?
Directed Energy: Remarkable Strides In Record Time
The U.S. Army wants you to know it’s not building a Death Star.** But what is happening – not just in the Army but across all branches of the military – is a billion-dollar annual investment in developing DE weapons concentrated on electromagnetic energy, according to the U.S. Government Accountability Office (GAO). “For example,” notes GAO, the Department of Defense (DOD) “has developed high-energy lasers that have successfully shot down drones in demonstrations. However, DOD has had trouble getting these technologies out of the lab and into the field for several reasons — including determining how to use them in missions.”
That’s not to say there haven’t been successes including the Army's Directed Energy Maneuver-Short Range Air Defense (DE M-SHORAD), a collaboration between the U.S. Army Combat Capabilities Development Command Aviation & Missile Center, the U.S. Army Space and Missile Defense Command and the Rapid Capabilities, Critical Technologies Office, and industry partners who built the next generation laser weapon.
“DE M-SHORAD is a 50-kilowatt class laser weapon system on a Stryker vehicle,” said Damon Templet, DEVCOM AvMC software lead for DE M-SHORAD. “In simple terms, we have an onboard thermal and power system that dissipates heat and recharges the system’s batteries. The laser is fired off the batteries.
“The beam comes out of a roof-mounted beam director. If you grew up on ‘Star Wars,’ it’s a little disappointing to learn that the beam makes no sound and isn’t visible. A tracking system puts the laser beam on a target and then maintains the optimal aimpoint until the track is neutralized.” The system is being continuously improved through Soldier touchpoints and live fire test events.
“I know how horrible it feels as a Soldier to watch enemy munitions fly over your position or explode over your head and there’s nothing you can do about it,” Templet said. “At a DE event a few years ago, young Soldiers told me about attempting to fire M-16s at enemy drones. DE provides an extra layer of protection to our Warfighters. We owe them that.”
** That’s a shame. How cool would a Death Star be?
Lasers For Communications In Low Earth Orbit
“With an eye on widespread internet access and defense, many space agencies and companies have zeroed in on laser-based systems for satellite-to-satellite communication,” writes All About Circuits. As a result, the field of satellite communication is experiencing unprecedented growth, and LEO satellites, in particular, have become increasingly popular. Traditional commercial satellites communicate between themselves and the ground using microwave or radio frequency signals. LEO constellations mostly talk with ground stations at microwave frequencies.
“The main purpose of these satellite ‘constellations’ is to improve and expand our telecommunications infrastructure,” writes Tech Briefs. “But, for constellations to offer the highest possible bandwidth transmission at the minimum cost, their satellites must be able to communicate directly through highspeed links. Laser-based transceivers offer one possible technological solution for these intersatellite links.”
When it comes to satellite communication, optical intersatellite links (OISLs) are a game-changer. OISLs offer faster and more reliable data transfer compared to traditional microwave or radio frequency (RF) communication methods thanks to the short distances between satellites and the lack of atmospheric interference in space.
Using a near-infrared laser beam, data is encoded and transferred through a small telescope to the recipient satellite, where it is detected and demodulated. What's more, the frequency of near-infrared light is in the hundreds of terahertz range, which supports substantially higher data rates compared to lower frequency microwaves.
This technology has long been used in terrestrial telecommunications, with near-infrared laser diodes transmitted through fiber optics. But for satellite communication, OISLs have incredible advantages over traditional methods. Not only do they offer faster and more reliable data transfer, but the lack of atmospheric interference means that they can be used for communication in low-earth orbit, geostationary orbit, and even far beyond.
“Near-infrared wavelengths also experience much lower diffraction compared to longer-wavelength microwaves,” Tech Briefs writes. “This allows a laser beam to be collimated and transmitted over a long distance without spreading much in terms of beam diameter. For example, a 1.55 μm wavelength laser with a 100 mm collimated beam diameter will only spread to 100 m in diameter over 5000 km. This can’t be accomplished with longer wavelength electromagnetic radiation like microwaves.”
The potential of LEO satellites is truly remarkable, and it's not hard to see why so many people are excited about the possibilities they offer. With improved optical and laser technology, we can expect to see even more impressive capabilities in the years to come
One Step Closer To Laser-Printed Medical Electronics
Lancaster University researchers led a team of scientists in developing a method to 3D print flexible electronics using the conducting polymer polypyrrole, according to the University. They have also shown that it is possible to directly print these electrical structures on or in living organisms (roundworms).
This process, still at the proof-of-concept stage, represents a major step towards 3D laser-printed materials that could be used in surgical procedures to implant or repair medical. It has the potential to print patient-specific implants for a variety of applications, including real-time health monitoring and medical interventions, such as treating epilepsy or pain.
“This approach potentially transforms the manufacture of complex 3D electronics for technical and medical applications – including structures for communication, displays, and sensors, for example,” said Dr. John Hardy, Senior Lecturer in Materials Chemistry at Lancaster University and one of the lead authors of the study. “Such approaches could revolutionize the way we implant but also repair medical devices. For example, one day technologies like this could be used to fix broken implanted electronics through a process similar to laser dental/eye surgery. Once fully mature, such technology could transform a currently major operation into a much simpler, faster, safer, and cheaper procedure.”
The two-stage study revealed how a high-resolution laser 3D printer, known as Nanoscribe, can be used to print electrical circuits within a silicone matrix. The researchers demonstrated how these 3D-printed electronics were able to stimulate mouse neurones.
The mouse brain tissue was kept alive in vitro, which allowed the electrodes to evoke neuronal responses similar to those seen in vivo, much like deep brain stimulation using neural electrodes. Dr. Damian Cummings, who led the brain stimulation work and works as a Lecturer in Neuroscience at University College London, has described how “readily customized implants for a wide range of tissues offer both therapeutic potential and can be utilized in many research fields."
The second stage of the study revealed how the process is compatible with living organisms, as conducting structures were 3D printed directly into nematode worms. Throughout the study, the ink formulations, laser exposure, and printing process were highly effective, producing exceptional results in the stimulation of neurones and conducting structures within organisms. The ability to use a 3D printer to create customized implants offers numerous possibilities for the medical community in many different fields, opening up new horizons of research and potential treatments.
The next steps in the development of research are already underway exploring the materials in which it is possible to print, the types of structures it is possible to print, and developing prototypes to showcase to potential end users who may be interested in co-development of the technology. The researchers believe the technology is around 10 to 15 years from being fully developed.