What Can Micro-Windmills Teach Us About The Future Of Medical Device Design?
By Jim Pomager, Executive Editor
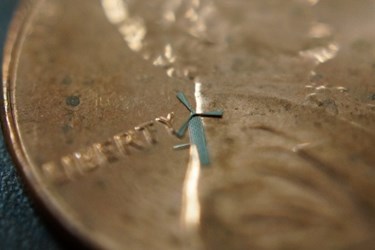
At some point in the not-so-distant future, you’ll be able to recharge your smartphone by waving it about in the air for a few moments or (less conspicuously) by simply holding it out in a stiff breeze.
Such is the intent of researchers at The University of Texas (UT) at Arlington, who have developed a micro-windmill that is 1.8 mm at its widest point and thinner than the diameter of a human hair — small enough that 10 could fit on a single grain of rice. Made of a unique nickel alloy, the windmills are also incredibly durable. If hundreds of them were affixed to a special sleeve, they could quickly and efficiently generate enough energy to restore power to a smartphone battery. The researchers are working with a Taiwanese foundry called WinMEMS Technologies to fabricate and commercialize the tiny machines.
What does this have to do with medical device design, you ask? Quite a lot, as it turns out. The micro-windmill design is one of many recent breakthroughs in the rapidly growing field of microelectromechanical systems, or MEMS, where miniaturized mechanical and electromechanical elements are married in devices that measure less than a millimeter in size. In addition to the windmill, the UT-WinMEMS collaboration has also developed MEMS gears, inductors, pop-up switches, and grippers, and foresees these miniscule devices as the building blocks of future micro-robots that will be used as (among other things) surgical tools, diagnostic probes, and other medical devices.
The UT at Arlington micro-windmill in action
MEMS have already arrived in several medical applications, and market research firms and industry associations alike believe that MEMS are poised for phenomenal growth in the sector. Transparency Market Research (Albany, NY) recently published a report showing that the global market for MEMS in medical applications was worth approximately $1.8 billion in 2012, and is expected to reach $6.5 billion by 2019. The French firm Yole Développement published very similar projections, forecasting the “bioMEMS” market (as they call it) to grow from $1.9 billion in 2012 to $6.6 billion in 2018.
“Tiny, intelligent MEMS sensors — more popularly known for enhancing the user experience with smartphones, tablets, and video game controllers — are improving medical technology in dramatic ways,” Karen Lightman, executive director of the MEMS Industry Group, said before the M2M Forum 2013. “From wearable and implantable drug-delivery systems to remote patient monitoring for diabetes and heart disease, medical researchers and technologists are collaborating on new applications that will improve patients’ health and quality of life in myriad ways.”
MEMS bring a wide range of advantages to medical device designs. One obvious benefit is that MEMS-based medical technology can have a significantly smaller footprint than traditional devices, and thus be less invasive. They can be mass produced using, in many cases, existing fabrication equipment, and many systems can be integrated onto a single chip. Power consumption is also typically lower for MEMS devices, meaning they can remain in the body or otherwise be used for longer periods of time without being recharged or replaced. Finally, they can enable far higher levels of accuracy, enabling the removal of very small amounts of tissue and the analysis of minute volumes of liquid samples.
As a result, the use of MEMS in medtech design is booming, particularly when it comes to sensing. Optical image sensors, pressure sensors, flow sensors, temperature sensors, biosensors, accelerometers, barometers, microphones, and host of other sensing technologies are either commercially available or are in development for medical use. MEMS are also enabling the creation of smaller components for radio frequency communication (RF-MEMS), microdispensing, microfluidics, energy harvesting, and other functions in medical design.
The following sections provide current examples of how MEMS are being leveraged by the design community to create innovative new medical devices and equipment for three burgeoning application areas.
Diagnostics: Handheld Retinal Scanner
Researchers at the Massachusetts Institute of Technology (MIT) have developed a new handheld ophthalmic-screening instrument that scans for diabetic retinopathy, glaucoma, macular degeneration, and other retinal diseases in a matter of seconds. Based on optical coherence tomography (OCT), the device incorporates an ultrahigh-speed 3D imager, a MEMS mirror for scanning, and a novel motion stabilization technique to correct for unintentional patient movement — enabling clinicians to test for multiple conditions with a single scan. The MIT group hopes the instrument’s portability will help extend eye screening beyond traditional points of care, and believes that the technology will ultimately find use in other medical applications, from surgical guidance to military medicine.
Therapeutics: Hollow, Inexpensive Microneedles
The University of British Columbia (UBC) is home to of a novel method for fabricating hollow MEMS microneedles. Much of the research into microneedles, patches of tiny needles used to deliver medication and vaccines without hitting nerves, has focused on solid needles that dissolve and release medication after puncturing the outer layers of skin. The UBC research, on the other hand, involves hollow cone- and pillar-shaped needles that are between 200 and 500 mm long and 50 and 80 mm in diameter. While early hollow microneedles were made of silicon, a material too expensive for widespread use, the UBC team is developing a cheaper, polymer-based design. In addition to removing the pain from injections, the plastic microneedles could eventually have applications in biosensing (e.g. blood-glucose monitoring).
Surgery: Minimally Invasive Surgical Instruments
A Boston University (BU) engineering professor is leading a project to use metal MEMS technology to construct instruments for intracardiac surgery, which can be used to remove tissue and perform repairs within the heart. Currently, the university is working with the Children’s Hospital Boston and Microfabrica to design devices and implants to close the patent foramen ovale (PFO), a congenital defect resulting in a valve-like opening between the two atria of the heart. The team is also developing a minimally invasive, joystick-controlled robotic instrument to accurately deliver the MEMS devices into a beating heart. When finalized, the technique is also expected to replace open heart surgery for a variety of other intracardiac procedures.
These case studies are but a small sampling of the advances being made by MEMS in the medical arena. While the technology is far from mature, it is clearly having a meaningful impact on the way new medical devices are being designed and built. Are there still challenges ahead for MEMS, before they can be fully embraced by the design community? Of course. But fabrication techniques, materials research (e.g. for biocompatibility), and equipment and processes development are progressing even as you read this. If you wait much longer to evaluate MEMS technology for your device design, the industry may have already moved on to the next, even-smaller evolutionary phase of development: nanoelectromechanical systems (NEMS).
For a comprehensive list of companies currently supplying and developing MEMS devices, materials, equipment, and services, visit the MEMS Industry Group’s searchable member capabilities database.
Image credit: University of Texas at Arlington News Center