Ultrafast Spectroscopy Reveals New Uses For Flawed Gems
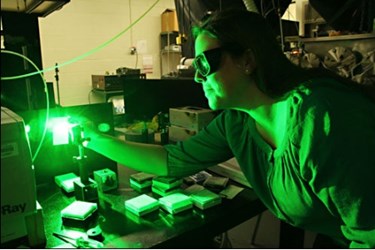
Ultrafast laser pulses have provided the first detailed observation of how energy travels through flawed diamonds. These flaws, known as nitrogen-vacancy centers, have interesting optical and electronic properties that are seen as promising for a variety of technological advances, such as quantum computing.
A team led by University of Arizona assistant professor Vanessa Huxter made the observation of how energy travels through diamonds containing nitrogen-vacancy centers – defects in which two adjacent carbon atoms in the diamond's crystal structure are replaced by a single nitrogen atom and an empty gap.
The findings could help scientists better understand the properties of the diamonds, and offer potential applications including the imaging of individual atoms in molecules.
Because the nitrogen-vacancy defects can be manipulated with optical methods such as lasers, they could be used for computing, data storage, sensing and even advanced imaging techniques capable of revealing the structure of molecules, said Huxter, who recently joined the UA’s department of chemistry and biochemistry. She led the research during a postdoctoral fellowship funded by the Natural Sciences and Engineering Research Council of Canada. Her team, which included Graham Fleming and Dmitry Budker at Lawrence Berkeley National Laboratory and the University of California, Berkeley, is the first to study the ultrafast dynamics in these crystals in real time.
"In order to use this system for these applications, we have to understand its fundamental properties," she said. "To use these systems for quantum computing, you want to have some idea of what we call vibrational modes, because they determine the local environment and may possibly be used for information processing."
Wherever a nitrogen-vacancy defect interrupts the gem's uniform carbon lattice, the vibrational properties change in ways that can be manipulated, for example by laser pulses.
"We use laser light to see what is happening in the system," Huxter said. "When we hit these things with ultrafast pulses, it's like hitting them with a hammer. We put a lot of energy into the system, and watch as that energy flows through it."
The laser pulse knocks the electrons in the nitrogen-vacancy centers into a higher energy level called the excited state. Over time, the electrons fall back into their ground state, in a process called relaxation, while dissipating the energy into their surroundings.
To watch how vibrations influence the ultrafast relaxation of the system, Huxter's team used ultrafast laser pulses, because the relaxation occurs on a timescale of a few nanoseconds.
Exactly how that energy moves through the crystal and how it influences the vibrations around the nitrogen-vacancy centers are crucial to figuring out how to take advantage of its properties, but nobody had ever been able to observe this process before.
"This is the first time we have been able to directly observe the vibrational spectrum of the system in real time," Huxter said.
The team employed two-dimensional electronic spectroscopy, basically a way of creating two-dimensional correlation "maps" that allow the researchers to watch the system as it relaxes to the ground state.
"Think of it as ultrahigh-speed photography to freeze the action on a scale of atoms and molecules," Huxter said. "We can watch the energy flow through the system in real time and take snapshots along the way. We can see where the energy is going in and where it is coming out."
They observed vibrations local to the defect with femtosecond time resolution. "Being able to directly follow these vibrations led to some surprising new results including that these vibrations are quantum mechanically coherent for thousands of femtoseconds," she said. "The question we ask is, what happens when you start replacing the atoms in the crystal? Will you get a change in the elastic properties?," she said. "Each nitrogen-vacancy center is like a softer region you can poke at. They absorb the laser energy where there was previously no absorption, and we see all these extra vibrational modes we don't see in the rest of the crystal. In our scenario, the diamond is like a clear window. We look straight through it and only see the defects. We tailor our laser pulse to the absorption of the defects." For more information, visit www.arizona.edu.
Source: University of Arizona