The Crucial Role Of Scientific Cameras In Biophotonics

By John Oncea, Editor
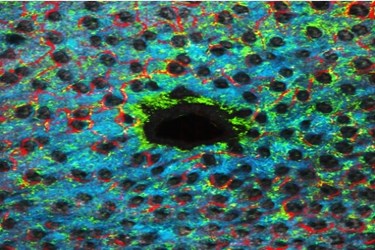
Scientific cameras are pivotal in biophotonics, enabling high-resolution imaging, spectral analysis, time-lapse observation, and quantitative analysis of biological processes from cellular to molecular levels. They empower diverse applications, from capturing dynamic events in microseconds to non-invasively studying biological structures, playing a critical role in advancing fields like medicine, biology, environmental science, and agriculture through their tailored functionalities for specific scientific needs.
Scientific cameras play a crucial role in biophotonics, the study of optical processes in biological systems that occur both naturally and in bioengineered materials. These cameras are designed to capture and analyze the light emitted by biological samples or tissues and are being used in several different ways.
Before we look at that, let’s take a minute to better define biophotonics.
Biophotonics 101
Biophotonics, according to AIP Publishing, is the study of biological objects using light in fields such as biology, medicine, pharmaceutical science, environmental science, and agriculture. This science operates on the principle that light can interact with biological objects through scattering, absorption, and emission. Additionally, biophotonics involves the development and application of optical technologies for these purposes.
The term itself dates back to the 16th century when the optical microscope was invented to visualize biological tissue. “Today,” AIP Publishing writes, “with the advent of superresolution fluorescence microscopy recognized by the 2014 Nobel Prize in Chemistry, cellular architecture, and function can be studied with outstanding spatial resolution down to 10 nm.”
Optical technologies play a crucial role in medical diagnosis and therapy in clinics and hospitals. They are used for early detection and prognosis of cancer through techniques such as endoscopy, flow cytometry, and fluorescence in situ hybridization (FISH). Similarly, ophthalmology relies heavily on optical coherence tomography (OCT) and laser-assisted in situ keratomileusis (LASIK) as powerful diagnostic and surgical tools.
Biophotonics play a key role in enabling diverse spatial and temporal scales, as well as improving invasiveness and functionality. Biophotonics is also highly practical and compatible and, as a result of all of this, “can generate tremendously large amounts and many types of biomedical data,” writes AOP Publishing. “The availability of biomedical Big Date has fueled the rapid advance of machine learning and its applications in biology, medicine, pharmaceutical science, environmental science, and agriculture.”
Biophotonics uses near-infrared light for various medical applications. Optical biopsy is a technique that uses near-infrared light to analyze tumor margins and lymph nodes, while optical mammography creates three-dimensional images of breast tissues. Another biophotonics technique is in vivo optical imaging, which is used in medical applications like functional brain imaging and optical breast cancer imaging.
Picture This
Scientific cameras are based on two sensor technologies: the original CCD-based cameras, including the EMCCD and ICCD, and CMOS cameras including sCMOS or scientific CMOS. Each type has different strengths and weaknesses making them suitable for specific applications, allowing us to “observe single photons in experimental physics and quantify single molecules in experimental biology,” writes Oxford Instruments. “We can view processes within whole small animals, experiment on organoids, and study the complexities of neural networks in the brain. We can watch chemical and biological processes on nanosecond timescales.”
When choosing between cameras, Oxford Instruments provides the following tips:
- sCMOS cameras are currently the most popular camera option for scientific applications due to their advanced engineering that allows for high performance in terms of speed, resolution, field of view, dynamic range, and sensitivity, without compromising on any of these aspects. These cameras find extensive use in various fields such as developmental biology, neurophysiology, solar astronomy, and dynamic X-ray imaging.
- CCD cameras are still the preferred choice for specialized applications that require a wide field of view, high dynamic range, and high sensitivity to capture weak signals over long exposure times which can span from minutes to hours. These applications include Astrospectroscopy, Astronomy, Neutron Radiography, luminescence, Plant Science Research, and Biochip reading.
- An EMCCD camera is highly sensitive and can detect even a single photon. It is particularly useful in applications that require exceptional sensitivity, such as fluorescence microscopy and super-resolution microscopy in life sciences, as well as Ultra Fast Spectroscopy, Single Molecule Spectroscopy, and Chemical Mapping in physical sciences. With its high speed, which operates on nanosecond timescales, it is an ideal tool for researchers in a variety of fields.
- ICCD cameras are commonly used for various scientific applications such as plasma studies, time-resolved fluorescence, photoluminescence, and Raman spectroscopy.
As noted, scientific cameras are essential for capturing, analyzing, and understanding biological processes at various levels, from cellular structures to dynamic molecular interactions. They facilitate a wide range of research, from basic biology to medical diagnostics and therapy development. Designed to be quantitative, they can reliably detect low signals and determine photon intensities via sensors that detect and count photons emitted from the sample.
How Scientific Cameras Are Being Used By Biophotonics
Biophotonics advancement has been driven in large part by the use of scientific cameras, specifically by the enabling of superior imaging and imaging of weak signals. Here are just a few of the ways they are used.
- Imaging: Biophotonics often involves imaging cellular or molecular structures using various light-based techniques such as fluorescence, confocal microscopy, or multiphoton microscopy. Scientific cameras are used to capture these images with high resolution and sensitivity. They can detect and record the emitted light signals from biological samples labeled with fluorescent markers or dyes.
- Spectral Analysis: Some biophotonic techniques involve analyzing the spectral characteristics of biological samples, such as the absorption or emission spectra of specific molecules. Scientific cameras equipped with spectral analysis capabilities help in capturing and analyzing this spectral data.
- Time-Lapse Imaging: Biological processes often unfold over time. Scientific cameras allow researchers to capture time-lapse images of dynamic biological events, such as cell division, movement, or reactions, enabling the study of temporal changes in biological samples.
- Quantitative Analysis: These cameras are equipped with software that allows for quantitative analysis of the captured images. This analysis might involve measuring fluorescence intensity, tracking movement, or quantifying changes in cellular structures.
- High-Speed Imaging: In some cases, biophotonic processes occur rapidly. High-speed scientific cameras can capture events that happen in microseconds or milliseconds, enabling the study of rapid physiological or cellular processes.
- Non-invasive Imaging: Biophotonics aims to develop non-invasive imaging techniques for studying biological systems. Scientific cameras are integral to these techniques, providing high-resolution images without damaging the samples.