Photonic Gap Band Materials: Engineering The Flow Of Light

By John Oncea, Editor
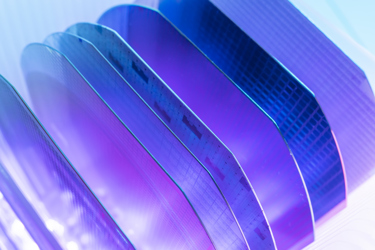
Photonic gap materials, or photonic crystals, are structures with a periodic dielectric profile that prevent light from propagating at certain wavelengths. This creates a "gap" where light cannot travel, offering potential for optical devices like filters and waveguides.
Formed in Tulsa, OK in 1967, the Gap Band was based around the Wilson brothers – Charlie, Ronnie, and Robert. The band’s name was a tribute to Greenwood, Archer, and Pine Streets in Tulsa’s historic Greenwood neighborhood. For me, the Gap Band peaked with 1982’s You Dropped a Bomb on Me, a song featuring a synthesizer that imitates the whistling sound of an aerial bomb being dropped.*
The Gap Band, according to AllMusic, didn’t enjoy a major commercial breakthrough until its third album. Some R&B lovers think that 1979's self-titled … hold on. I’m being told this is supposed to be an article about band gap materials, not the Gap band.
Never mind.
* This was the 80s, and that kind of stuff was OK.
Subhead
Since the advent of semiconductor physics, engineers have mastered the control of electron flow using band gap theory. Similarly, photonic band gap (PBG) materials, or photonic crystals, offer the capability to manipulate the propagation of light.
PBGs, also known as photonic crystals, are materials that have a periodic structure on the scale of the wavelength of light, which affects how photons propagate through them, according to ScienceDirect. These periodic structures influence photon behavior, enabling engineers to selectively block or transmit specific wavelengths. As photonics continues to evolve, PBG materials are becoming instrumental in applications ranging from telecommunications to quantum computing.
PBGs are composed of a range of light frequencies (or wavelengths) that cannot propagate through the material. This occurs due to the periodic variations in refractive index, which cause destructive interference for certain wavelengths. The periodicity can be in one, two, or three dimensions:
- 1D photonic crystals: Multilayer dielectric mirrors, such as Bragg reflectors.
- 2D photonic crystals: Arrays of holes or rods etched in a substrate, guiding light in specific planar geometries.
- 3D photonic crystals: Structures like woodpile lattices or diamond-like networks that block light in all directions and polarizations.
PBG applications include optical fibers and waveguides with low loss, controlling spontaneous emission in lasers and LEDs, optical filters, and mirrors, and developing cloaking materials and optical computing.
In these materials, light waves of certain wavelengths are reflected or inhibited from propagating due to the repeated interaction with the material’s internal structure, similar to how electrons are forbidden from occupying certain energy bands in semiconductors.
The Physics Of Photonic Band Gaps
A photonic band gap refers to a range of optical frequencies where electromagnetic waves are prohibited from propagating through a material. This phenomenon arises from destructive interference caused by periodic variations in the refractive index, akin to how a crystal lattice in a semiconductor restricts certain electron energies.
The periodic structure induces Bragg scattering of light. When periodicity matches the wavelength of light, specific frequencies undergo multiple reflections and cancel out, forming a band gap. Photons within these forbidden frequencies are either reflected or attenuated, unable to traverse the material.
The concept of photonic band gaps was independently introduced in 1987 by Eli Yablonovitch and Sajeev John, who proposed manipulating light using periodic dielectric structures. Yablonovitch suggested that certain dielectric materials could inhibit spontaneous emission, a concept pivotal to enhancing LED and laser efficiency. Concurrently, John explored light localization phenomena, with implications for disordered media.
These foundational ideas have since evolved, with significant contributions from researchers like John Joannopoulos, who has extensively studied photonic crystals' ability to control light propagation.
Engineering Photonic Crystals Today
Advancements in high-resolution fabrication, computational design, and material characterization have propelled photonic crystal engineering forward. Bragg reflectors, composed of alternating dielectric layers with varying refractive indices, are prevalent in devices like vertical-cavity surface-emitting lasers (VCSELs), filters, and sensors. Their fabrication is relatively straightforward and often integrated during epitaxial growth processes.
2D photonic crystals are integral to integrated photonic circuits. Typically, a silicon slab is perforated with a periodic array of holes. Introducing a line defect – by omitting a row of holes – allows light to be guided through the crystal with minimal loss. These structures are foundational in advanced waveguides, optical switches, and cavity resonators used in dense photonic integration platforms.
3D photonic crystals can confine light in all directions, offering comprehensive control over photon flow. Fabricating these structures remains challenging, but recent breakthroughs have demonstrated the feasibility of 3D printing photonic crystals with complete band gaps in the visible spectrum using customized titanium resins.
Applications In Photonics Engineering
The unique properties of PBG materials enable a range of practical applications, including low-loss optical waveguides. PBG waveguides can confine light with exceptional precision, minimizing scattering losses even around tight bends. This capability is crucial for compact on-chip photonic networks and signal processing.
Next is enhanced light-matter interaction in which, by confining light in ultra-small volumes with high Q-factors, photonic crystals enhance interactions with embedded emitters or nonlinear materials. This is particularly beneficial in quantum information processing, sensing, and low-threshold lasers. Other applications include:
- Optical Filters and Mirrors: High reflectivity within specific wavelength ranges makes PBG structures ideal for narrowband filters, tunable mirrors, and wavelength-selective devices in fiber optics and lasers.
- LEDs and Laser Control: Photonic crystals can suppress or enhance spontaneous emission at specific wavelengths, improving LED efficiency and enabling directional emission in laser cavities.
- Cloaking and Metamaterials: Combining PBG structures with metamaterials can lead to exotic optical behaviors, such as cloaking or negative refraction.
- Optical Computing and Signal Routing: PBG-based devices hold promise for all-optical switching, logic, and memory operations—key steps toward optical computing.
Despite their advantages, PBG materials face several challenges, including fabrication complexity because achieving high-precision fabrication at sub-wavelength scales, especially in 3D, remains difficult and costly.
In addition, PBG materials are sensitive to disorder, and minor defects in periodicity can cause scattering and degrade performance; photonic band gaps are often narrow, limiting their flexibility in broadband applications; and merging photonic crystals with conventional electronics and packaging presents significant engineering hurdles.
Ongoing research and advancements in fabrication techniques are gradually addressing these limitations.
Emerging Directions And Future Potential
The future of photonic band gap materials lies in multidisciplinary innovation, with topological photonics being one key area of development. Inspired by condensed matter physics, topological photonic crystals offer robust light transport modes that are immune to scattering from defects or disorders, an exciting avenue for resilient photonic circuits.
Programmable and active photonic crystals – the incorporation of materials responsive to electrical, thermal, or optical stimuli allows for tunable photonic band gaps – is a second emerging direction. This tunability paves the way for adaptive optics, reconfigurable circuits, and dynamic filtering.
Finally, 3D photonic crystals with embedded quantum dots or defects are poised to play a pivotal role in quantum computing, enabling deterministic single-photon sources and photon-photon gates, while self-assembly techniques could significantly reduce the cost and complexity of fabricating 3D photonic crystals, making them viable for commercial use.
A Transformative Moment
Photonic band gap materials represent a transformative development in photonic engineering. By enabling precise control over light propagation, they unlock powerful capabilities in communication, computation, sensing, and energy.
For engineers, mastering these materials means entering a realm where light can be designed, confined, and directed with the same precision that electrical engineers apply to electrons. As fabrication techniques mature and theoretical understanding deepens, the applications of photonic crystals will continue to expand, ushering in a future where photons are the cornerstone of next-generation technologies.