Fiber Optic Cables: How Far Is Too Far?

By John Oncea, Editor
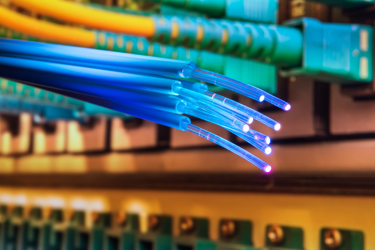
With ideal conditions and amplification, optical fiber can transmit petabit speeds globally, but real-world limits depend on fiber type and network design.
Given perfect conditions in a lab-like setting without ensuring no signal degradation, how far could fiber optics transmit data? Hundreds of miles? Thousands?
Well, we don’t live in a lab-like setting. In real-world scenarios, factors like fiber quality, equipment limitations, and signal processing introduce limitations, making such long distances impractical without amplifiers.
For instance, without amplifiers, single-mode fiber can reach 50-60 miles and can support data rates of 1 Gbps or 10 Gbps. With amplifiers, such as Erbium-doped fiber amplifiers (EDFAs), the distance can be extended to 600 miles or more, and even further with additional amplifiers for long-haul applications.
The reach of multimode fiber, which has a larger core diameter and supports multiple modes of light propagation, is significantly shorter. For example, OM3 multimode fiber can support 10 Gbps over 325 yards, and OM4 can support it over 420 yards. At lower data rates, multimode fiber can reach just over a mile.
What Are Fiber Optic Cables
Fiber optic cables are advanced communication cables that transmit data as pulses of light, rather than electricity, through extremely thin strands of glass or plastic known as optical fibers, according to Fluke Networks. Each fiber is about the diameter of a human hair and can carry vast amounts of information at very high speeds, making fiber optic technology fundamental to modern telecommunications, the internet, and data networks.
The basic structure of a fiber optic cable includes several layers. At the center is the core, made from ultra-pure glass or plastic, where light signals travel. Surrounding the core is the cladding, also made of glass or plastic, which has a lower refractive index. This difference in refractive indices causes the light to reflect internally, ensuring it remains within the core as it travels down the cable, The Network Installers write. Outside the cladding, a protective buffer or coating absorbs shocks and prevents damage, while an outer jacket shields the cable from environmental hazards.
Fiber optic cables come in two main types: single-mode and multimode. Single-mode fiber has a very thin core (about 8–9 microns in diameter) and transmits a single light signal, making it ideal for long-distance communication with minimal signal loss. Multimode fiber has a larger core (typically 50 or 62.5 microns) and can carry multiple light modes, which is suitable for high-speed data transmission over shorter distances.
Compared to traditional copper cables, fiber optic cables offer several advantages. They support much higher data rates and bandwidth, are immune to electromagnetic interference, and can transmit data over longer distances without significant signal degradation, writes Hosa. Additionally, fiber optic cables are lighter, more durable, and do not conduct electricity, making them safer and more dependable in various environments.
These properties make fiber optic cables essential for high-speed internet, cable television, telephone services, and many industrial and medical applications, forming the backbone of global digital communication networks.
12-Mile Cables: Russia’s Unjammable Drones
We earlier reported that Russia has turned to fiber optic drones in response to Ukraine’s advance into the Kursk region. Instead of using radio to transmit controller data to the drone and video data from the drone, all communication takes place over a fiber optic cable that is spooled out from the drone as it flies.
No longer susceptible to jamming or loss of radio signal, the drone is now limited only by its battery life. The drone also can operate past usual distances, through terrain such as trees that would usually block the signal, at much lower to the ground, and in areas covered by electronic weapons. It’s like a low, slow TOW missile.
“Its operation is ingenious and simple: the drone has a built-in reel with a cable, which can be between three and 15 miles long,” writes EL PAÍS. “If, during flight, the cable becomes entangled in an obstacle, such as trees, the vehicle continues flying because the reel keeps releasing it, and it remains connected to the pilot.”
One notable aspect of Russia’s use of fiber-optic drones is that highly trained units operate them. This is evident in Kursk and at another location on the front line, specifically in the battle for Chasiv Yar in the Donetsk region.
These drones are piloted by skilled teams that can reach Ukrainian artillery positions located 6.2 miles from the enemy. “They can even fly between tree lines to determine if our howitzers are present; unfortunately, they are causing many casualties,” reports Andriy Horetskiy, commander of a battery in Ukraine’s 26th Artillery Brigade.
There are other benefits, too. The latency for a video feed to pilot the drone is going to be extremely low and not affected by terrain, allowing for much more aggressive flying. The drone’s antenna can be used for signals intelligence, and it can pass a large spectrum of frequencies across that fiber, something not possible via RF.
As interesting as that may be, the takeaway here is that fiber-optic drones are limited to about 12 miles. How much further can we push the limits of fiber optics?
Pushing The Limits
The maximum effective distance a fiber optic cable can work depends on several factors, including the type of fiber, the quality of the cable, the data transmission rate, and the use of signal amplification technologies.
The effective range of a fiber optic link is fundamentally determined by attenuation, chromatic and modal dispersion, optical power budgets, and the cumulative effects of splices and connectors.
Attenuation remains a primary constraint: as light pulses propagate through the fiber, they are gradually absorbed and scattered by the glass matrix. In modern low-loss single-mode fibers, attenuation rates can be as low as 0.16 dB/km at the 1550 nm window, allowing signals to travel over 60 miles before the optical power drops below detectable thresholds for standard receivers.
With advanced amplification and dispersion compensation, single-mode fiber can reach even further, routinely supporting transmission distances of 100–200 kilometers in real-world deployments, and even more in specialized long-haul or undersea applications, Linden Photonics writes.
For multimode fiber, which is typically used for shorter distances within buildings or campuses, the maximum range is much less. OM1 multimode fiber supports up to 325 yards at 1 Gbps, OM2 up to 650 yards, OM3 up to 325 yards at 10 Gbps, and OM4 up to 600 yards at 10 Gbps, according to Show Me Cables. These shorter distances are due to modal dispersion, where multiple light paths within the fiber cause signal spreading and limit the maximum transmission range.
Factors Limiting Transmission
Several key factors influence how far a fiber optic cable can transmit data effectively. Attenuation, or the reduction in signal strength as light travels through the fiber, is caused by inherent material absorption, scattering, and losses at splices or connectors.
Lower attenuation allows longer transmission distances. Chromatic dispersion, where different wavelengths of light travel at slightly different speeds, causes the signal to spread out and potentially degrade over long distances. Optical power, including higher input power and more sensitive receivers, can extend the transmission distance, as the signal remains detectable over greater lengths.
Higher bandwidth signals are more susceptible to dispersion and attenuation, which can limit the maximum achievable distance. Each splice or connector introduces some loss, which can accumulate and reduce the maximum effective distance, writes Twinkle Fiber.
Amplification technologies, particularly erbium-doped fiber amplifiers (EDFAs), have dramatically extended the reach of optical systems. By boosting the optical signal without electrical conversion, EDFAs enable transmission over hundreds of miles between regeneration sites.
In modern dense wavelength division multiplexing (DWDM) systems, spans of 50-65 miles between amplifiers are standard, and total system reaches of 635 miles or more are routine in backbone networks. The use of Raman amplification, which leverages distributed gain along the fiber itself, can further increase span lengths and reduce noise figures.
Recent advances in multicore fiber and space-division multiplexing (SDM) are pushing the boundaries of what is physically possible. In 2024, Japanese researchers reported a record-breaking experiment transmitting 389 terabits per second over 630 miles of multicore fiber, with each spatial channel maintaining high data rates and signal integrity, NTT reports.
While such systems are not yet commercially widespread, they illustrate the direction of future ultra-long-haul and high-capacity networks, where spatial and spectral efficiency are maximized through sophisticated photonic design and signal processing.
System Guidelines
In practical, commercially deployed networks, the following guidelines are typical: single-mode fiber can reliably carry data up to 60-120 miles before requiring amplification, and with in-line amplifiers and dispersion management, this range can be extended to over 620 miles, as seen in many national and transcontinental backbone networks.
Submarine cables, which must traverse thousands of miles, rely on carefully spaced optical repeaters, typically every 40 to 60 miles, to maintain signal quality across oceanic distances. Each repeater site is a marvel of photonic engineering, balancing gain, noise, and reliability over decades of operation.
Data rate is another crucial variable. As transmission speeds increase, the effects of dispersion and nonlinearity become more pronounced, reducing the maximum achievable distance for a given system design.
For instance, while 10 Gbps signals can travel hundreds of miles with appropriate dispersion management, 400 Gbps and higher rates require more frequent amplification and tighter control of dispersion and nonlinear effects2. This tradeoff between capacity and reach is a central challenge in the design of modern optical networks, driving ongoing research into advanced modulation formats, digital signal processing, and new fiber types.
In summary, fiber optic cables are capable of transmitting data over impressive distances, with single-mode fibers routinely covering up to 120 miles in real-world applications, and even longer distances with advanced technologies. Multimode fibers, while limited to much shorter ranges, are still essential for high-speed connections within buildings and campuses. The ongoing evolution of fiber optic technology continues to extend these limits, supporting the ever-growing demands of global communication networks.
Theoretically Speaking …
With no practical limitations, the maximum distance and speed at which optical fiber cables could transmit data are determined by the fundamental physics of light transmission, the quality of the fiber, and the sophistication of transmission and amplification technologies.
The theoretical speed of data transmission in optical fiber is governed by two main factors: the speed of light in glass and the channel bandwidth. Light in optical fiber travels at about two-thirds the speed of light in a vacuum, or roughly 125,000 miles per second.
However, the more relevant measure for data capacity is bandwidth. Modern research has demonstrated that, using advanced techniques such as wavelength-division multiplexing (WDM) and geometric constellation shaping, a single optical fiber can transmit up to 178 terabits per second (Tbps) – a figure approaching the Shannon limit for fiber communication, according to Tom's Hardware.
Some theoretical estimates suggest that, with perfect conditions and unlimited wavelengths, the upper limit could be in the range of petabits per second per optical mode, StackExchange writes. In practice, commercial systems are currently far below this ceiling, but the technology continues to advance rapidly.
In theory, light could travel through fiber indefinitely, but signal attenuation and dispersion limit practical distances. With ideal amplification and signal regeneration, there is no hard upper bound to how far data could travel-transoceanic cables already span over 6,200 miles using repeaters.
The only real constraint is the ability to maintain signal integrity and manage noise over extreme distances. In a perfect scenario with unlimited, lossless amplification and perfect error correction, data could be transmitted around the globe at these extreme speeds.
Given no real-world limitations, optical fiber could transmit data at speeds in excess of 178 Tbps, potentially reaching petabit-per-second rates, over any distance-including global scales-so long as perfect amplification and regeneration are available.