Design Case Study: Fiber Optics Simplify Terahertz Imaging and Spectroscopy, Part I
By: J.V. Rudd, Picometrix Inc. and Colin Seaton, <%=company%> Group
For more than a decade now, a growing number of researchers have been catching their first glimpse of the world in the terahertz neighborhood of the spectrum. Located between the microwave and infrared spectral regions, this virtual backwater of electromagnetic frequencies from about 0.1 to 10 THz had languished for years in relative obscurity because terahertz radiation was so difficult to generate and detect. As a result, the optical and spectroscopic properties of many materials remain uncharacterized in this spectral range today.
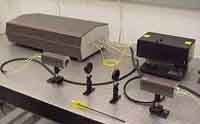
The situation began to change in the 1990s with the development of novel optoelectronic technologies for producing and detecting terahertz energies (also known as T-rays), including ultrafast lasers, nonlinear optical effects, and advanced semiconductor physics. These new tools have inspired a variety of innovative spectroscopic studies of semiconductors, liquids, gases, and even flames at terahertz frequencies.
T-ray systems have even been used to monitor the water content of leaves, spot defects and voids in circuit boards, and find the raisins in a sealed box of cereal. But it is the potential for commercial applications such as screening luggage for plastic explosives that has prompted some to describe T-rays as the most significant breakthrough since X-rays.
Standing in the way of commercialization, however, is the complexity of terahertz technology itself. T-ray systems are usually built and operated by researchers and scientists who have an intimate understanding of the physics behind the instruments, which makes them less accessible to those who do not. Picometrix, Inc. (Ann Arbor, MI) has confronted this problem by developing a compact, computer-controlled T-ray system based on technology originally conceived at Murray Hill, NJ-based Lucent Technologies (see photo at top). Coherent Inc., Laser Group (Santa Clara, CA) produced the compact, turnkey laser and grating dispersion compensator (GDC) used to drive the system.
The central features of the new instrument are its hermetically sealed, fiber-pigtailed modules for transmitting and receiving terahertz radiation and its software control of hardware, data collection, and image processing. These attributes make T-rays much more accessible to the general research community and signify a crucial advance toward commercial use.
Terahertz time-domain spectroscopy
The basic principle underlying the new system is terahertz time-domain spectroscopy (THz-TDS), a pump-probe method pioneered by researchers at AT&T Bell Laboratories and IBM's T. J. Watson Research Center. THz-TDS avoids many of the problems that normally plague spectroscopy in the far infrared by using a train of ephemeral T-ray pulses approximately 1 ps in duration. Each pulse contains just one cycle of terahertz radiation and is created by an even shorter pulse of laser radiation about 100 fs in duration. The key to this technique lies in how the T-rays are created and detected.
In the T-ray system, the femtosecond output of a modelocked titanium-doped sapphire (Ti:sapphire) laser is split into two beams (see Figure 1). One beam, called the pump, leads directly to a T-ray emitter (transmitter). The other beam, called the probe, leads to a T-ray detector (receiver) via a variable delay line.
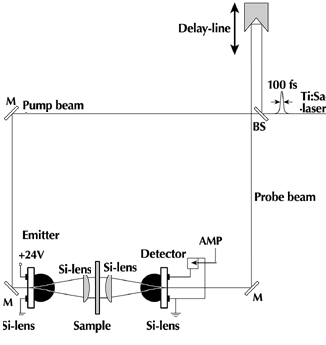
At the transmitter, the pump-beam pulses are focused onto a photoconductive semiconductor wafer with a tiny dipole antenna structure lithographically fabricated onto its surface. A dc bias of several tens of volts applied across the gap between the antenna arms, which measures only a few microns, creates a high electric field. Each laser pulse absorbed by the semiconductor material within the antenna gap generates a swarm of charge carriers (electron-hole pairs) that are strongly accelerated by the electric field. The accelerating charges produce a single-cycle burst of terahertz energy that radiates outward from the semiconductor surface.
A hyperhemispherical lens made of high-resistivity, low-dispersion silicon (Si) collects the radiation, and additional optics collimate the beam into a diffraction-limited T-ray.
T-ray detection
After passing through (or reflecting from) the sample being analyzed, the T-ray is directed onto a receiver similar in design to the transmitter. A hyperhemispherical Si lens focuses the T-ray pulse into the antenna gap on the photoconductive semiconductor surface, and the laser probe pulse enters from the other side. Unlike the transmitter, the receiver antenna is not externally biased. However, if the T-ray and probe pulses arrive simultaneously, the electric field amplitude of the terahertz pulse drive charge carriers generated by the probe pulse.
An amplifier measures the resulting antenna current imprinted with the transient T-ray waveform. Essentially, the probe pulses synchronously and repetitively gate the receiver, thereby transforming it into a boxcar averager with sub-picosecond resolution. Performing a Fourier transform on the time-domain T-ray pulse yields the terahertz frequency spectrum, which reflects any contributions made by the sample.
The fundamental advantages of THz-TDS over conventional techniques originate from its coherent transmission and detection schemes. For example, T-rays can be focused, collimated, transmitted, and reflected just like laser beams, therefore images can be constructed by scanning the object. THz-TDS is also extremely sensitive, with signal-to-noise ratios (SNR) of 10,000:1. Coherent, gated detection avoids the need for cryogenic cooling, too, because the receiver rejects nearly all of the incoherent radiation, which means T-ray systems can operate in ambient light and at room temperature.
With so many advantages, it is easy to understand why THz-TDS has garnered such attention from researchers throughout the world. It is also easy to appreciate the challenge of building a commercial T-ray instrument. Aside from the state-of-the-art ultrafast laser system required, a T-ray instrument places stringent conditions on optics, optical alignment, mechanical stability, environmental control, semiconductor manufacture, electronics, and signal analysis.
In Part II of this feature, the authors describe a T-ray system developed for commercial applications.
Acknowledgements
Special thanks to Dave Zimdars and Matt Warmuth at Picometrix Inc. for their contributions to the development of the T-ray system, and to the Air Force SBIR program and Program Manager J.R. Gord for partially funding our work.
About the authors…
J.V. Rudd is terahertz project leader at Picometrix Inc., PO Box 130243 Ann Arbor, MI 48113-0243. Phone: 734-998-4501; fax: 734-998-3474. Colin Seaton is business development and marketing manager at Coherent Inc. Laser Group, 5100 Patrick Henry Dr., Santa Clara, CA 95054. Phone: 408-764-4983; fax: 408-988-6838.