Amplification Of Relativistic Electron Pulses By Direct Acceleration In The Laser Field
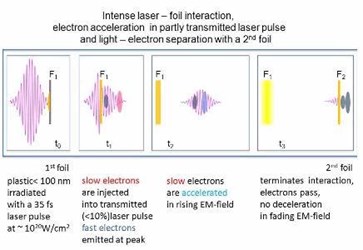
A targeted and direct acceleration of electrons in extremely strong laser fields would enable novel, ultra-compact accelerators to be realized. However, to achieve this goal, the intrinsic motion of electrons in the electromagnetic alternating field of a laser pulse must be rectified and decoupled from the field. This fundamental challenge is intensively explored worldwide. In experiments at the Max Born Institute, researchers have now succeeded in realizing a concept of direct laser acceleration and, theoretically, in detail. This concept opens up the possibility of generating relativistic and ultra-short electron pulses at extremely small acceleration distances below one millimeter. Such electrons and X-ray sources based on them have a wide range of applications in spectroscopy and structure analysis, in medical-biological research and in nanotechnology.
How electrons in very strong laser fields can be accelerated to relativistic energies touches a fundamental question of the physics of light-matter interaction. Although a free, resting electron is driven by the electric and magnetic fields of a laser pulse to oscillations at extremely high velocities, the electron is quiescent again with the decay of the light field and a net energy transfer by the direct acceleration in a laser field does not take place. This basic principle, which is often discussed in physics tests, however, is bound to certain prerequisites for the spatial extent and intensity of the laser pulse. However, if these conditions are violated, eg by focusing the laser or the presence of strong electrostatic fields in a plasma, electrons can actually be accelerated by the interaction with a laser pulse.
Many research groups are currently working on the question of the speed at which electrons can be extracted from an extremely strong laser field and how short ultra-short laser pulses can be used to generate correspondingly short electron pulses of high charge density.
In a light field with a "relativistic" intensity (I> 1018 W / cm²), the electrons oscillate at speeds close to the speed of light and their kinetic energy is in the range of megoelectron volts (MeV) to gigaelelectronvolts (GeV at I> 1022 W / cm²). These strong light fields can be achieved by focusing very short laser pulses with high pulse energies on the area of a few micrometers. The resulting spatial intensity distribution already allows an acceleration of electrons to high kinetic energies. The principle is known as "ponderomotive" acceleration and represents an elementary process in the interaction of strong light fields and matter. Various theoretical studies have predicted that the number and energy of the electrons can be significantly increased by an additional direct acceleration in the laser field , But only when the electron-light interaction is deliberately interrupted. These considerations were the starting point for the experiments of Julia Braenzel and her colleagues at the Max Born Institute.
In the experiments at the MBI, the electrons were decoupled from the light pulse at a very specific time by means of a separator film which is impermeable to the laser light. Thus it could be shown that the number of electrons can be increased at high velocities. With a 70 TW Ti: Saphir laser (2 J @ 35 fs) and 30 to 100 nm thin target films made of PVF plastic, <109 electrons could be generated with kinetic energies in the MeV range, which by the ponderomotive force in the propagation direction of the laser Were emitted. During the actual interaction, the film is in an almost fully ionized state, that is, it has become a plasma.
For sufficiently low film thicknesses below 100 nm, a part of the laser light can pass through this plasma, and the electrons already emitted behind the film are thereby overtaken by the transmitted light pulse. Quasi-intrinsically synchronized, the "slow" electrons are injected into the transmitted, still relativistic laser field (<8 x 1018 W / cm2). If a second thin film is then placed as a separator at a suitable distance behind the first film, a gain of the electron signal can be determined in a very specific energy range. Figure 1a shows schematically the temporal sequence in the experiment and in Figure 1b the resulting electron distribution is compared, which is obtained without and with additional separator film. The separator film is impermeable to the transmitted laser light but is permeable to the fast electrons, so the electrons can be decoupled from the light field. The time at which the interaction of the electrons with the transmitted laser pulse is interrupted is determined by the distance between the foils.
The experiments carried out in the group by Matthias Schnürer show that the amplification of the electron signal becomes maximal at a certain distance and disappears completely for very large distances. The theoretical concept of leaving electrons by a decoupling of the laser pulse in time after acceleration to high kinetic energies was confirmed by numerous series of measurements and numerical simulations. The experiments and the analytical model show that slow electrons with kinetic energies below 100kV are accelerated to about one order of magnitude higher energy by the presence of the second film. This effect leads to densification of the electrons in a narrow energy range. In contrast to the so-called "keel wave acceleration", which has already demonstrated the generation of GeV electrons with a laser-driven plasma wave, the direct laser acceleration can be scaled to very high laser intensities and plasma radii. In addition to basic physical insights, future applications in the field of laser-based sources of relativistic electrons also arise on the basis of this concept.
Source: Forschungsverbund Berlin e.V.