6 Microscopy Stories 10 Minutes

By John Oncea, Editor
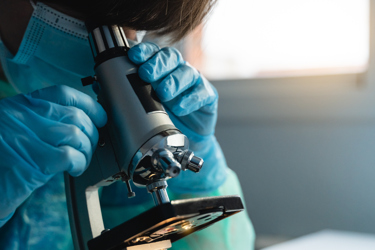
Inspired by a radio show inspired by a theater troupe, we look at six microscopy-related stories in what Google tells me should take 8.3 minutes to read. Your mileage may vary.
July 11, 2003: An estimated 364,146 babies were born; “The Sopranos” actress Jamie-Lynn Sigler weds her manager A.J. Discala at Brooklyn Botanical Garden in New York; four bored Fairfield, CA teens confessed to making a dozen or so crop circles that mysteriously appeared in a wheat field, and This American Life aired “20 Acts in 60 Minutes.”
“Instead of the usual ‘each week we choose a theme and bring you 3 or 4 stories on that theme’ business, we throw all that away and bring you 20 stories—yes, 20—in 60 minutes,” writes This American Life. “Inspiration for this week’s show came from the Neo-Futurists, whose long-running Chicago show promises 30 plays in 60 minutes every single weekend.”
So, inspired by a radio program that was inspired by “an evolving, multi-faceted theatrical aesthetic built on a belief in truthful, direct communication between the performer and the audience,” I present to you six stories about microscopy in, depending on how fast you read, 10 minutes.
New Year, New Opportunities
We start with four trends to be aware of as we work our way through 2024 courtesy of Wiley Analytical Science: vEM, CLEXM, FIB, and AO.
Wiley asserts last year was a big year for Volume Electron Microscopy (vEM) with the user community steadily growing, especially in the UK. vEM growth was bolstered by several high-profile publications including Nature’s Volume EM: a quiet revolution takes shape.
Nature “documented the technologies that underpin the approaches using electron microscopy including serial sectioning in an SEM directly using an ultramicrotome, serial sectioning and collection of the sections to a tape or other support, and use of Focused Ion Beam Scanning Electron Microscopes (FIB-SEM) for repeated removal of material followed by image collection.”
Nature’s article raised awareness of ways vEM is being applied, as well as “the need to not only build the infrastructure to perform the microscopy but also the need to train experts in this area.”
While vEM is a relatively new technology, Correlative Light and Electron Microscopy (CLEM) is now well-established as a concept. “An emerging idea is the addition of X-Ray microscopy data to the light and EM microscopy, in an attempt to add further information, imaging throughput, and context,” writes Wiley. “Details of exactly how this is best done are still to be optimized, but an EU-funded project aims to combine soft-x-Ray microscopy in multimodal disease research.”
The Marie Sklodowska-Curie Doctoral Networks Action (MSCA-DN) is a project funded by the European Union under Horizon Europe. The network is composed of seven partners and last year welcomed its first cohort of nine Ph.D. students. According to the project’s website, the main objective of the CLEXM project is to provide advanced training in the field of correlative multimode imaging to a new generation of doctoral candidates. This will equip them with the necessary skills to pursue successful careers in an area of high growth that can help researchers better understand diseases and develop effective treatments. We are eagerly anticipating the early results of the next iteration of CLEM.
Wiley also addresses cryo-plasma FIB, writing, “In recent years, the Plasma Focused Ion Beam has made a massive impact on the FIB-SEM community in its ability to remove huge volumes of material to enable fields of view hundreds of micrometers.”
Researchers in the life sciences have been using Xenon plasma and other species to visualize cells and tissues in frozen material, replacing the more traditional resin-embedded protocols. Similarly, materials scientists are using a combination of sample cooling and FIB to study battery materials in the presence of their liquid electrolytes without the need to remove them. We anticipate that this field will continue to grow, sparked by the release of microscope platforms from Tescan and ThermoFisher.
Finally, Wiley writes, “A machine learning-based approach from UK-based researchers at the University of Oxford looks to provide a universal adaptive optics (AO) solution that can be readily transferred between microscope modalities.”
The researchers have successfully tested their method on multiple types of microscopes, including two-photon, three-photon, and widefield 3D structured illumination microscopes. Their results have shown better performance than sensorless AO methods. Analysis indicates that their method outperforms conventional methods by correcting aberrations with fewer sample exposures and with robustness to challenging imaging tasks, such as coping with high noise levels, random sample motions, and blinking events in functional imaging.
Targeting Human Brain Pathology
A new variant of expansion microscopy that is tailored for brain pathology samples reveals cells and structures that can’t be seen with other methods, writes Chemical and Engineering News (C&EN). Expansion microscopy is a technique that allows an ordinary diffraction-limited optical microscope to achieve the equivalent of super-resolution. In this technique, tissue samples labeled with fluorescent markers are embedded in a gel and treated with an enzyme that breaks down the proteins. The sample is then expanded equally in all directions, which leads to the proteins being pulled apart while still maintaining the same relative positions to one another.
“Edward S. Boyden of the Massachusetts Institute of Technology, E. Antonio Chiocca of Harvard Medical School, and coworkers call their new expansion microscopy variant decrowding expansion pathology, or dExPath,” writes C&EN. “In dExPath, instead of digesting the proteins, the researchers’ process uses a softening buffer that keeps the proteins intact.
“An initial partial expansion begins the process of separating the proteins. Because the proteins are still intact, they can be labeled with fluorescently labeled antibodies after the decrowding step, which results in improved labeling density and image quality.”
The researchers utilized dExPath for multiplexed imaging of different human brain pathology samples, including those from patients diagnosed with glioma, Alzheimer’s disease, and Parkinson’s disease. By removing overcrowding, researchers were able to identify cell populations or structures that were previously undetected through conventional immunostaining. This staining process can be repeated multiple times on the same tissue to visualize several markers on the same tissue.
Detailed Images Of Brain Cancer Tissue
A recent study by researchers from Brigham and Women’s Hospital has introduced a new microscopy technology called decrowding expansion pathology (dExPath). This technology has enabled them to produce unprecedentedly detailed images of brain cancer tissue. Their findings offer new insights into the development of brain cancer, with potential implications for the diagnosis and treatment of aggressive neurological diseases.
“In the past, we have relied on expensive, super-resolution microscopes that only very well-funded labs could afford, required specialized training to use, and are often impractical for high-throughput analyses of brain tissues at the molecular level,” said Pablo Valdes, MD, Ph.D., a neurosurgery resident alumnus at the Brigham and lead author of the study. “This technology brings reliable, super-resolution imaging to the clinic, enabling scientists to study neurological diseases at a never-before-achieved nanoscale level on conventional clinical samples with conventional microscopes.”
Their new technology chemically modifies tissues by embedding them in a gel and ‘softening’ the tissues with a special chemical treatment that separates protein structures without destroying them and allows tissues to expand. This provided exciting findings to the MIT and Brigham researchers, who routinely use commercially available antibodies to bind to and illuminate biomarkers in a sample. Antibodies, however, are large and often cannot easily penetrate cell structures to reach their target. Now, by pulling proteins apart with dExPath, these same antibodies used for staining can penetrate spaces to bind proteins in tissue that could not be accessed before expansion, highlighting nanometer-sized structures or even cell populations that were previously hidden.
While promising, dExPath requires validation on larger sample sizes before it can contribute to the diagnosis of neurological conditions such as brain cancer. Valdes underscores that, although still in its early stages, his team aspires for this technology to eventually serve as a diagnostic tool, enhancing patient outcomes.
“We hope that with this technology, we can better understand at the nanoscale levels the intricate workings of brain tumors and their interactions with the nervous system without depending on exorbitantly expensive lab equipment,” said Valdes who is now an assistant professor of neurosurgery and Jennie Sealy Distinguished Chair in Neuroscience at the University of Texas Medical Branch. “The accessibility of dExPath will enable super-resolution imaging to understand biological processing at the nanometer level in human tissue in neuro-oncology and neurological diseases such as Alzheimer’s and Parkinson’s, and one day, could even improve diagnostic strategies and patient outcomes.”
A Center Of Excellence
Leica Microsystems and the Department of Biochemistry at the University of Oxford have recently collaborated to establish a Centre of Excellence for advanced microscopy, reports The Analytical Scientist. The Micron Bioimaging Facility at Oxford will host the center, which will serve as a platform for experts to share their knowledge through workshops and seminars.
“We aim to accelerate scientific discoveries and inspire the next generation of scientists,” said Darin Stell, Senior VP Global Commercial Operations at Leica Microsystems.
The Analytical Scientists poke with Naomi Wills, Head of Microscopy Sales in the UK, and James O’Brien, Vice President Life Sciences and Applied Solutions, both at Leica Microsystems to learn more, covering everything from what sparked the collaboration and the new center’s aim to the state of microscopy today and what to look for in 2024.
Measuring Up
A ruler is commonly used to measure everyday objects, as it has a fixed length and marked divisions. Scientists at Julius-Maximilians Universität (JMU) Würzburg in Germany have developed a new device called PicoRuler that follows the same principle but can measure tiny objects such as cells and molecules, reports Physics World.
This minuscule measuring stick can work in biological environments and is useful for testing the ability of super-resolution microscopy techniques to image objects less than 10 nm in size. Over the past two decades, super-resolution microscopy has rapidly advanced, and fluorescence imaging-based techniques can now resolve structures as small as a few nanometers, below the diffraction limit of conventional visible-light microscopy.
“To push these techniques further, researchers need reference structures to calibrate their microscopes’ performance,” Physics World writes. “The main calibration method currently in use relies on artificial DNA origami structures. These can be synthesized to carry several fluorophores at well-defined positions less than 10 nm apart, allowing them to act like rulers for sub-10 nm imaging. The problem is that DNA origami is highly negatively charged and thus cannot be used in real-world biological cellular imaging media.”
The team of researchers is currently working toward optimizing their ruler for use in various biological environments, such as living cells. According to Sauer, one of the directions for further development could be to directly deliver PicoRulers into the cells themselves using techniques like microinjection or functionalization with cell-penetrating peptides. This would enable scientists to explore a cell's structure from within, providing invaluable knowledge that could advance cellular biology and lead to a better understanding of diseases and pathways for drug development.
Groundbreaking Held For Ground-Breaking Microscope
In a groundbreaking move, Jennifer Schisa’s Biosciences laboratory has propelled itself into the forefront of scientific discovery with the acquisition of a specialized Zeiss Apotome 3 microscope system, made possible by a generous $100,000 supplemental grant from the NIH, writes Central Michigan University. The installation of this state-of-the-art fluorescence microscope marks the beginning of an exciting chapter, as researchers and students eagerly embark on a journey into uncharted territories of cellular structures and protein dynamics.
Cutting-edge technology has revolutionized the possibilities within the lab by expanding the scope of visualizable fluorescently-tagged proteins and boasting high-resolution imaging capabilities comparable to confocal microscopes. What sets this technology apart is its user-friendly design, making it accessible to students who can actively engage in data collection to finalize a groundbreaking publication. The advanced technology has opened the door to answering new questions as researchers explore the intricate world of proteins, unhampered by previous technological constraints. The newfound capabilities of the microscope have enabled students to undertake experiments that were previously impossible.
Researchers aspire to understand if the formation of unusual protein granules indeed results in low-quality eggs, with the ultimate goal of paving the way for new therapeutic interventions to enhance fertility in women. As the studies progress, the researchers plan to compile their findings into a manuscript for submission in the coming months, anticipating that their discoveries may shape the future of reproductive biology.