Has Giant Space Spectrometer Found Evidence Of Dark Matter?
By Jim Pomager, Executive Editor
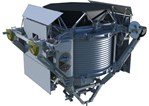
First of all, I feel I should apologize for all the astrophysics coverage here recently. (Last week's column discussed the Plank space telescope's mapping of light that dates back to the Big Bang.) It's not exactly a booming application area, and I know that few of you actually work in the space. However, it's hard to pass up a fascinating story (or two) when you find one, especially when photonics technology plays a leading role.
Back in May 2011, an international team of scientists watched as the $1.5 billion, 9-ton Alpha Magnetic Spectrometer (AMS) that many of them had spent the last 17 years of their life building and testing was carried from Earth aboard the space shuttle Discovery (on that craft's final mission) and bolted to the outside of the International Space Station (ISS). Soon after, a battery of detectors on the so-called "Hubble Space Telescope of cosmic rays" began gathering data on the thousands of particles that pass through it each second, enabling the instrument to begin fulfilling the purpose for which it was built — to find antimatter and dark matter and measure cosmic ray composition and flux. From this data, the researchers hope to gain a better understanding of, among other things, the universe's origin and evolution.
The Alpha Magnetic Spectrometer (AMS) on the International Space Station
Nearly two years later, the initial results are in. Last week, lead investigator Samuel Ting (1976 Nobel laureate and Thomas D. Cabot Professor of Physics at MIT) presented findings based on the approximately 25 billion events recorded by the AMS during its first 18 months in orbit. Most notably, Ting reported that the spectrometer had detected evidence of what could be dark matter, the mysterious, invisible substance scientists hypothesize makes up a significant portion of the universe's total mass and energy.
Now I'm no particle physicist, but I'll do my best to quickly explain how the AMS works, what it found, and why it's so important...
The AMS measures cosmic rays, high-energy particles that travel throughout space. It's better qualified to perform this task than bigger and more powerful earth-bound spectrometers due to its location outside the atmosphere, which absorbs most cosmic rays. As cosmic particles pass through the aperture of the AMS, a huge magnet bends their path through a series of detectors that includes:
- Transition Radiation Detector (TRD) – identifies electrons and positrons (which we'll get to in a minute) among other cosmic rays
- Time of Flight System (ToF) – records transit time of the particles through the AMS (think really fast stopwatch) and warns sub-detectors of incoming rays
- Silicon Tracker – measures the charge of particles, distinguishing between matter and antimatter
- Ring Image Cerenkov Counter (RICH) – calculates the velocity of particles
- Electromagnetic Calorimeter (ECAL) – differentiates between protons and positrons and measures their total energy
- Anti-Coincidence Counter (ACC) – rejects unwanted particles and saves useful ones for analysis
These sensors are incredibly fast (particles pass them by in nanoseconds), extremely precise/accurate (capable of identifying one part in 10 billion), and able to gather enormous amounts of data (1 GB/s). The information they collect is regularly transmitted via the ISS communication systems to teams of AMS physicists (located in 16 different countries) for analysis.
The project's main quarry is the aforementioned positron, the antimatter equivalent of the electron. (Positrons have the same mass and magnitude of charge as electrons, but are positively charged.) During its first year and a half of operation, the AMS recorded 400,000 positrons, the largest collection of antimatter particles ever recorded in space. AMS researchers observed an excess of positrons in the cosmic ray flux consistent with the "supersymmetry" theory, which speculates that positrons could be produced when two particles of dark matter collide with and annihilate one another.
Why all the fuss over dark matter? Based on anomalies observed in the gravity and rotation of galaxies, astrophysicists have posited that there must be much more to the universe than ordinary matter —everything from what we see with our naked eye down to elementary particles such as quarks. Ordinary matter may account for no more than 5% of the total mass-energy of the universe. The other 95%? You guessed it: dark matter and its cohort dark energy. That's a lot of mostly uncharted scientific territory.
Dark matter presents a tantalizing-yet-elusive prey for scientists, since it doesn't emit light or absorb electromagnetic radiation at any meaningful level. As a result, its existence thus far has been more inferred than observed. The AMS team aims to change all that.
Next up, AMS scientists will look to substantiate their initial findings, which were far from definitive. For instance, the antimatter excess observed by the AMS could have originated from pulsars, neutron stars that emit electromagnetic radiation, rather than dark matter collisions. Lead investigator Ting is confident an answer could come soon: "Over the coming months, AMS will be able to tell us conclusively whether these positrons are a signal for dark matter, or whether they have some other origin."
The good news is AMS team should have at least 7 more years to sort out this and other mysteries of astrophysics. The instrument will remain in operation until either the ISS project runs out of cash (ISS is currently funded through 2020, and could be supported through 2028) or its magnet fails (estimates range from 10 to 18 years). Until then, we can celebrate this initial triumph of spectroscopy and wait to see whether the technology and the information it uncovers will challenge the way we think about the laws of physics and the origins of the universe.
Images and video courtesy of NASA